Putting the Nature of Science strand into the water cycle
Chris Joyce, Ally Bull, Rosemary Hipkins, and Bill MacIntyre
Understanding how water is recycled and redistributed from place to place is important for sustaining the health of our planet. Developing solutions to issues such as global warming, climate change, water usage, growing more food, and protecting wildlife is dependent upon this understanding. The water cycle is, therefore, an important context for school science.
However, to be able to use knowledge about the water cycle for making decisions, students need to understand that the water cycle is a complex and dynamic system in which multiple relationships impact on each other.
Traditionally, the water cycle has been taught as a simple system. This is supported by the uncritical use of diagrams, the function of which is to simplify ideas. In fact, attempts to simplify may not only lead to misconceptions about the water cycle, but also do not encourage students to engage with the messiness of a complex system.
Communicating in science
The new science curriculum represents a change of approach for teachers as they plan for their science teaching. Instead of starting with a science “topic” (such as the water cycle) or a science “big idea” (e.g., water can be a solid, a liquid, or a gas), teachers are asked to begin with a Nature of Science objective, and the science content strands become the context for exploring this. This does not mean that the science knowledge, skills, and understandings are regarded as unimportant. In fact, we argue that this change of starting point can lead to an enhanced understanding of the science.
Communicating in science is one of the four achievement aims in the Nature of Science strand. It is closely aligned to the key competency, Using language, symbols, and texts. The water cycle provides a very rich context for addressing Communicating in science. Because the processes can take place over a large area (planet Earth), and because we cannot see aspects of some of the processes (such as evaporation and condensation), we often use diagrams and models to represent what is happening, not just in order to communicate but also as an aid for developing understanding. While other elements of Communicating in science will be touched on, the main focus of our research, and therefore this article, is the use of representations such as drawings, diagrams, and models.
The research
There is a large body of existing research literature on students’ understandings of evaporation and condensation, including the Learning in Science Project (LISP) at Waikato University (Osborne & Freyberg, 1985) and Science Processes and Concept Exploration (SPACE) in Britain (Russell & Watt, 1990). This was our starting point for further research to inform the development of the Assessment Resource Banks (ARBs) and other New Zealand Council for Educational Research (NZCER) resources.
The NZCER research comprises four related fields of enquiry:
•&&Systems thinking. This investigation was part of the ongoing research that informs the design of science resources for the ARB, and was based on some work by Assaraf and Orion (2005). The context was waterways. From this research, four stages of systems thinking were identified: identifying the parts of a system; identifying links between parts of a system without explaining the relationship; describing direct relationships; and describing multiple relationships that impact on each other (Bull, Joyce, & Hipkins, 2007a). This research provided a useful framework for considering students’ understandings of the water cycle, because this is also a system.
•&&Students’ understandings about the water cycle. This was another investigation to inform ARB resources. A representative sample of 655 students from Years 4–10 from 17 New Zealand schools completed written items designed to find out their understandings about aspects of the water cycle (Bull, Hipkins, Joyce, & MacIntyre, 2007a).
•&&Students’ representations of changes of state. Several resources trialled with students at Year 4 and Year 10 (again as part of the ARB research) were designed to investigate how students represented changes of state.
•&&Using language, symbols, and texts. Classroom trials were carried out for a game and teacher resource for developing this key competency through the context of the water cycle (Bull, Hipkins, Joyce, & MacIntyre, 2007b).
Understanding the science
From all these pieces of research, we are able to draw several conclusions about students’ understandings of the water cycle.
The water cycle is a system. The model we developed for identifying stages in students’ thinking about waterways can also be applied to the water cycle. Students have to know about the “parts” or elements of a water cycle. These include water in its various states, processes such as evaporation and condensation, and theoretical ideas used to explain relationships, such as particle theory. They also need to know about direct relationships between parts; for example, when the sun heats the water it evaporates. However, the water cycle needs to be understood as a complex and dynamic system. If we want students to be able to use their knowledge of the water cycle to communicate and make decisions, they have to be able to recognise multiple relationships that impact on each other; for example, higher temperatures raise sea level by expanding ocean water, melting mountain glaciers, and causing land-based ice sheets to melt.
New Zealand students’ initial understandings of the “parts” of the water cycle, such as the processes of evaporation and condensation, were comparable to those described in the research literature (summarised in Tables 1 and 2). Over time (measured in terms of the year level of the students rather than the progress of individual students), in most areas students’ understandings become more closely aligned with the scientific explanations. In the case of evaporation, a common pattern in the ARB research was for a big jump in student understanding between Years 4 and 6. There was little progress in understanding between Years 8 and 10, despite the fact that at Level 5 the 1993 curriculum suggested introducing particle nature of matter to investigate the different states of familiar substances (such as water). At Year 10 a large number of the trial students still retained misconceptions, or did not provide sufficient information in their responses for a teacher (or researcher) to make a valid judgement about their understanding.
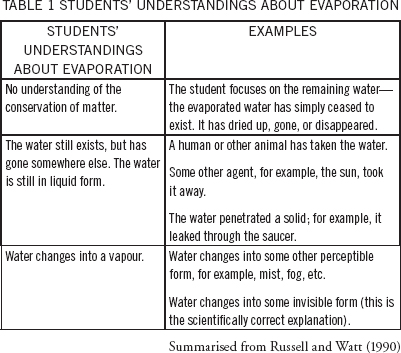
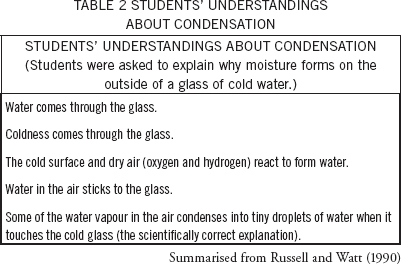
The gaseous state of water is particularly challenging for students. There is compelling evidence that even when they talk about water vapour, many of them retain misconceptions because they cannot imagine water being invisible. It is very common to muddle steam and water vapour, not recognising that once we can see the water (as it becomes steam) it is a mixture of water vapour and liquid water.
When students learn a new piece of knowledge, they often describe inappropriate relationships as they try to reconcile the new idea with their existing understandings. An example from our research is the concept of temperature being a driver for evaporation. Years 6–8 students were more likely than younger or older students to include temperature in their explanations for why the water level stayed the same in a closed jar but did not in an open one—even though it was an irrelevant factor in this situation.
Implications for the classroom
Because there are many elements to the water cycle, it is beneficial to students’ learning if they revisit this system throughout their time at school, so more “bits” can be added or understandings adjusted as they explore increasingly complex contexts.
Students have to know about the processes that drive the water cycle, but they should also explore the relationships between the various elements. Some examples of simple relationships are: temperature is a factor that influences the state water will be in; wind transports water vapour from where it evaporates to where it eventually rains; and (for older students) heat energy is stored in oceans.
More complex and “real-life” situations help uncover misconceptions students hold. They also challenge students to rethink their explanations, because they are no longer adequate for the new situation. As students explore more complex contexts, they can appear to be more confused than they were before they started (Bull et al., 2007a). This can be unsettling for some students (and their teachers!) because they have to be able to tolerate uncertainty as they try to adjust their theories. However, other students are motivated by this challenge, and tolerating uncertainty is known to be an important disposition to foster for 21st-century learners (see, for example, Claxton, 2007).
Adding the Communicating in science layer
Through working with teachers in classrooms and investigating students’ responses to ARB items, we have identified a number of advantages to be gained when, when teaching the water cycle, the Nature of Science strand, Communicating in science, is used as a focus. In this section we will discuss two types of representation commonly used by scientists, and also used in classrooms to aid understanding and thinking. These representations are models and diagrams, and the focus will be on their classroom use. We will then briefly discuss scientific vocabulary, and the importance of talk as a learning tool.
Interpreting and using models
Models are a kind of representation often made use of in both science and science education. In science teaching, models are frequently employed to demonstrate or investigate a particular aspect of the water cycle. Although our data showed that older students are more likely to be able to interpret a model, in general this did not seem to be a very highly developed skill. Some areas of difficulty were:
•&&recognising that a model represents something real, but is not the real thing
•&&recognising what each part of a model represents
•&&comparing a model with the real thing
•&&using a model to justify their explanations.
Particle theory can be used as a model for thinking about the dynamics of the water cycle. Because many of the water cycle processes are invisible they can only be explained satisfactorily at a particle level. When Year 4 and Year 10 students were asked to draw what water looked like as it evaporated from a puddle (Figure 1), as expected almost all students at Year 4 drew a macroscopic view (Johnstone, 1993), that is, the water was no longer visible, or, in some cases, they showed with labels or wavy lines that the water (vapour) went into the air. What was more surprising, however, was that at Year 10 only 19 students in the sample of 294 drew the process of evaporation as a submicroscopic view, that is, they drew how the arrangement of particles changed. Those students who did so were able to demonstrate that they had a very clear understanding of the way the water particles behave.
Comments from the older students were interesting. While most Year 4 students enjoyed the drawing task, a number of the Year 10 students disliked the task, either because they “did not like drawing” or because they believed they couldn’t draw something invisible.
Implications for the classroom
Time taken to explore what a model is representing is time well spent, as it helps unpack the science understandings. A strategy using models for teaching is to have students analyse the similarities and differences of the model and the real thing it is modelling. This helps them appreciate what aspect of reality the model is representing, and where the model breaks down. It is important to keep the science focus (e.g., evaporation, or the water cycle) to the forefront as this analysis is carried out.
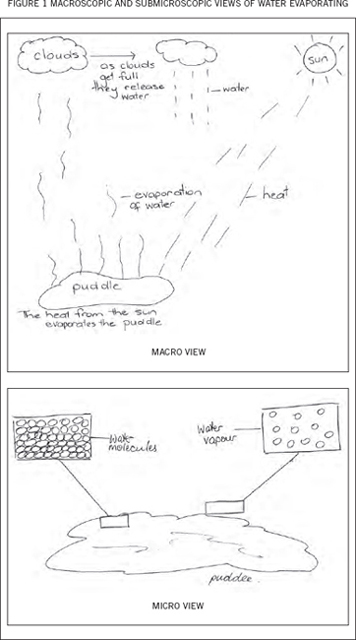
An important part of helping students to understand particle theory is to explore how it is represented by scientists, and to encourage them to represent their own ideas in drawings.
Water cycle diagrams
The water cycle diagram is a special sort of model. We know that students are generally quite skilled at reproducing a water cycle diagram. However, very few of the students from the `ARB trials were able to add all the arrows to a diagram that represented the water cycle differently from how it is usually presented (Figure 2).
This was likely to be partly due to their not understanding the science (the misconceptions were exposed by having to complete the unfamiliar diagram), but there was evidence to suggest that there are also difficulties with understanding the conventions used in a water cycle diagram. There were three main difficulties:
•&&The ocean at the top and the clouds at the bottom of the diagram caused confusion for many students, especially younger ones. These students are likely to be unaware that diagrams are a representation of a particular idea, not a drawing of reality.
•&&Some students made the arrows form a full circle around the outside of the diagram. These students seem to think that a cycle should literally form a circle.
•&&All the arrows added pointed “downhill”, that is, in the direction that rain would fall if this were taken literally.
Implications for the classroom
Figure 3 is an example of a typical water cycle diagram used in primary schools. At secondary level the diagrams used may be a little more complex, but are, in many cases, essentially similar.
This sort of diagram, in an attempt to be more user friendly, tends to blur the boundaries between the real and the symbolic. Hence we get a picture of a landscape (itself a type of representation of reality), and superimposed on this are elements of a diagram such as arrows and labels. The intention is to make things clearer for the reader. But does it really? Students still need to be able to interpret the “shorthand” of a diagram to be able to make sense of it.
If we look at this diagram literally, what does it imply? It seems to say that the water goes around in a circle, that it is a set cycle with no variations, and that there are some processes (evaporation, infiltration, transpiration, and precipitation) involved. It also implies that the water evaporates from the lakes and streams (often diagrams just show the ocean) and falls on the land. We can see water vapour.
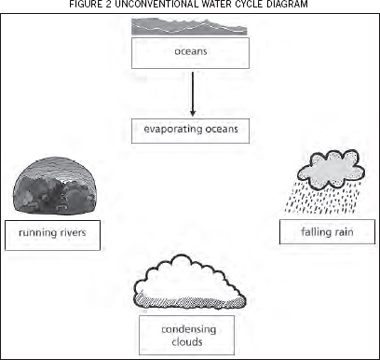
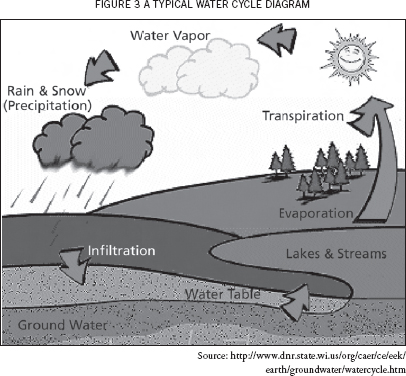
Evaporated water appears to go to the sun. If students don’t know how the symbolism of this diagram is intended to work, there is plenty of potential for their reinforcing misconceptions or even developing new ones.
Reading or creating a water cycle diagram involves using a combination of science knowledge and the conventions of diagrams. Students need to know not only about the processes of the water cycle, but also that:
•&&only part of the story is shown in the diagram
•&&some parts of the diagram show some things that you cannot really see (e.g., the invisible water vapour is shown in Figure 3 as a smudge, and we cannot see at a particle level how water changes from liquid to vapour and back again)
•&&the diagram does not show some things that are important
•&&a cycle is not a circle; it shows the order the processes may occur (although in fact there may be cycles within the water cycle)
•&&the processes are represented by arrows.
It is also important to be aware of the writers’ purpose when they created the diagram, so students know what aspects of the water cycle are being shown in the diagram. When students draw their own diagrams they should be encouraged to consider their purpose in doing so, and the most effective way to present their ideas.
Science vocabulary and talking
As students learn scientific language, they are more likely to use the scientific words without unpacking what is actually involved. For example, when asked what happens to the water when it disappears from a swimming pool, they respond that it evaporated. This does not provide any indication of the ideas they have about what this entails.
Talk is another form of communication. Observations in classrooms and feedback from teachers have confirmed that activities that encourage students to share and justify their ideas help them to form their own theories. Students challenge one another and, in justifying their position, they either strengthen their position or recognise weaknesses in their ideas. Another advantage is that teachers can often pick up and address students’ misunderstandings from their conversations.
Implications for the classroom
A focus on key scientific language encourages students to understand the meaning of the words they are using, rather than just incorporating them into their vocabulary without understanding the concept. Word games such as “Loopy” (Bull et al., 2007b) and “Matching” are enjoyed by students and are effective in developing their vocabulary.
Group activities can be effective conversation starters, for example: producing a concept map of the water cycle; group responses to questions such as, Where has the water gone?; and playing games.
Conclusion
To summarise, learning about the water cycle is challenging because:
•&&one state of water is invisible
•&&the processes that drive it are largely invisible (although we can see the results in the form of dried up puddles, rain, frost, clouds, etc.)
•&&an understanding of particle theory is necessary to fully understand the way the water behaves
•&&the water cycle is a complex system and so many different factors may be interacting at any one time
•&&many traditional representations of the water cycle misrepresent it as a “simple” rather than complex and dynamic system
•&&students need to understand a number of communication conventions to read and write about their own and others’ ideas about the water cycle.
The water cycle is complex to understand and complex to teach. However, it is also essential knowledge for appreciating its role in the health of our planet. If we want students to work towards developing deep understanding of a complex and messy system, they have to be able to do more than reproduce a water cycle diagram. We want them to be able to use what they know to make sound decisions about the world they live in.
Our research affirms that knowledge is important—knowing about the parts of the water cycle system is essential. However, this knowledge of parts, by itself, is insufficient. It is also important to understand that there are simple interactions between these parts so students can begin to understand that different interactions impact on each other. It is when we investigate these complex interactions that how the “parts” impact on the outcomes becomes less certain. Also important is recognising that both human activity and natural occurrences, such as a major volcanic eruption, can impact on the water cycle system. In addition, knowing about how science ideas are communicated can enrich students’ understanding of science. Discussing what is represented in a diagram, picture, or model helps students make meaning of the various representations. Being aware of the conventions used not only makes the science more accessible, but also provides a toolbox for students to communicate their own ideas, not just in science, but in other areas as well.
Incorporating Communicating in science into learning about the water cycle can not only enrich students’ understandings of the science concepts but it also provides opportunities for them to both evaluate others’ ideas and present their own effectively. This provides a platform for them to make meaningful decisions as they use their knowledge in their everyday lives.
References
Assaraf, O., & Orion, N. (2005). Development of system thinking skills in the context of Earth system education. Journal of Research in Science Teaching, 42(5), 518–560.
Bull, A., Hipkins, R., Joyce, C., & MacIntyre, B. (2007a). What are New Zealand students’ ideas about changes of state of water and the water cycle? Retrieved 25 June 2008, from http://arb.nzcer.org.nz/supportmaterials/science/water_cycle
_research.php
Bull, A., Hipkins, R., Joyce, C., & MacIntyre, B. (2007b). Key competencies: The water cycle: A science journey: Teacher’s notes. Wellington: NZCER Press.
Bull, A., Joyce, C., & Hipkins, R. (2007). Thinking about the “big picture”: How can school science contribute? set: Research Information for Teachers, 1, 15–20.
Claxton, G. (2007). Expanding young people’s capacity to learn. British Journal of Educational Studies, 55(2), 115–134.
Johnstone, A. H. (1993). The development of chemistry teaching: A changing response to changing demand. Journal of Chemical Education, 70(9), 701–705.
Osborne, R., & Freyberg, P. (1985). Learning in science: The implications of children’s science. Auckland: Heinemann.
Russell, T., & Watt, D. (1990). Evaporation and condensation (Primary Space Project Research Report). Liverpool: Liverpool University Press.
Chris Joyce is manager of assessment at the New Zealand Council for Educational Research.
Email: chris.joyce@nzcer.org.nz
Ally Bull is a senior researcher at the New Zealand Council for Educational Research.
Email: ally.bull@nzcer.org.nz
Rosemary Hipkins is a chief researcher at the New Zealand Council for Educational Research.
Email: rosemary.hipkins@nzcer.org.nz
Bill MacIntyre is a senior lecturer in science education at Massey University, Palmerston North. His research interests include assessment, curriculum integration, astronomy education, and pedagogical content knowledge.
Email: W.R.MacIntyre@massey.ac.nz